Phase Separation of Hydrogen
Motivation
The phase separation of hydrogen is a current research theme in the field of space technology which is of special interest concerning two different applications in future cryogenic propulsion systems: The gas or vapor free delivery of the liquid propellant to the combustion chamber on one side and the liquid free gas expulsion on the other side. Phase separation can be realized using the retention capability of a screen or double screen against liquid. Both applications are conceivable with autogenic pressurisation in a one species two phase system consisting of liquid hydrogen and hydrogen vapor as well as pressurisation with a non-condensable gas in a two species two phase system consisting of liquid hydrogen and gaseous helium.
Objectives
In this proposal a test facility has been developed which allows to analyse the physical phenomena related to the retention capability of a double screen against liquid hydrogen during ground and drop tower tests. The development of the test facility requiered first numerical test predictions which have been generated with the commercial CFD-software FLOW-3D. Using the test facility and the numerical test predictions an experimental campaign consisting of 14 ground and three drop tower tests in microgravity has been accomplished succefully.
Theory
Consider an outer glass cylinder partly filled with liquid hydrogen in hydrogen vapor environment like illustrated in Fig. 1. Inside the outer glass cylinder an inner glass tube is immersed coaxially into the liquid. Inside the inner glass tube a double screen is housed in a certain distance to the liquid-vapor interface. The double screen consists of a lower screen and an upper screen which are positioned coaxially to the outer glass cylinder and the inner glass tube with a certain constant space between each other. Applying a pressure difference between the vapor phases liquid rise can occur. If the meniscus wall contact line at the inner glass cylinder reaches the lower screen radial inward wicking can occur. The initial liquid free pores of the screen get imbibed by the liquid and form small menisci at the liquid vapor interfaces. Due to the curvatures of the small menisci capillary forces attacking at the interfaces move the liquid front radial inward to the center of the screen. At the end of the process the initial dry screen is saturated by liquid. In dependence of the liquid rise velocity and the radial inward wicking velocity a certain volume of vapor can be enclosed below the lower screen after saturation. If the enclosed vapor volume doesn't block the whole screen cross section in flow direction a leakage flow through the outer sides of the lower screen can occur. Because the leakage flow crosses the lower screen parallel to its thickness a screen crossflow pressure loss occurs. Therefore the liquid rise in the space between both screens becomes slower. If the meniscus wall contact line reaches the upper screen a second radial inward wicking process can take place similar to the first one. After saturation by liquid of the initial dry upper screen a second vapor volume can be enclosed below the screen, blocking a part of the screen cross section. Due to a leakage flow at the sides of the screen a second crossflow pressure loss can occur, slowing down the liquid rise above the upper screen and possibly stop at a certain height. In this case a phase separation between the liquid phase and the vapor phase above the upper screen is achieved.
The whole process depends on the differential pressure applied between the vapor phases at the outer glass cylinder and the inner glass tube. If the pressure difference exceeds the bubble point pressure the enclosed vapor volumes can be suppressed through the screens and the blocked cross sections below the screens become smaller. In addition to that phase change phenomena can overlay the process because a single species two phase system consisting of hydrogen liquid and hydrogen vapor is treated.
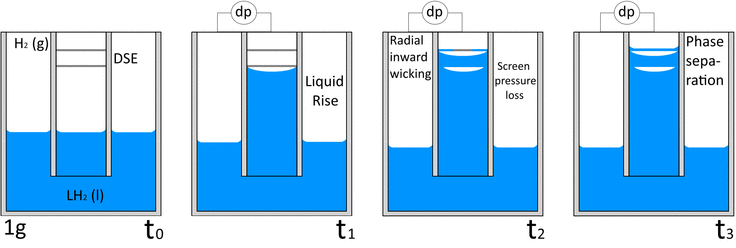
Numerical predictions
Numerical test predictions for the proposal described exemplarily in Fig. 1 have been generated using the commercial CFD-software FLOW-3D. In the top row of Fig. 2 the numerical results at certain time steps of the incompressible, isothermal, cylindrical and two dimensional simulation of a ground test is shown. In the lower row of Fig. 2 the associated numerical results of the drop tower test are presented. As can be seen the predicted governing physical phenomena liquid rise, radial inward wicking, screen crossflow pressure loss and bubble point pressure have been considered in combination for a DTW 200x1400 metal screen. The fluid is liquid hydrogen at saturation conditions. The liquid phase is red colored while the vapor phase is colored in blue. Phasechange phenomena have not been considered just for now. However with the aid of the test predictions the cryogenic test facility has been developed and accomplished successfully.
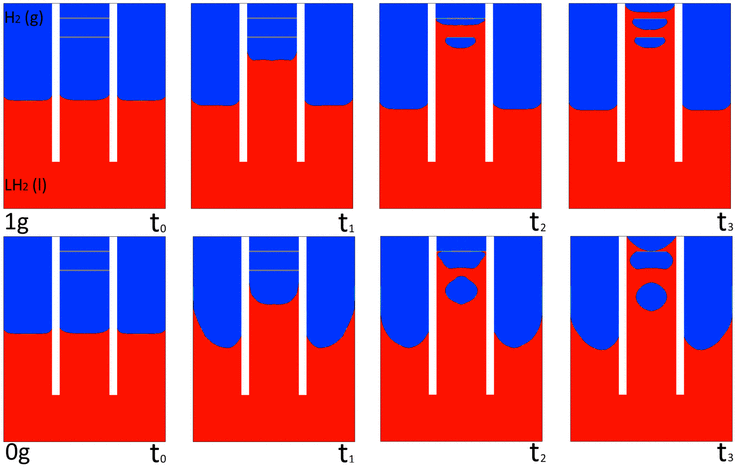
Experiments
An experimental campaign consisting of 14 tests in earth gravity and three drop tower tests in microgravity using the drop tower at the University of Bremen has been conducted.
With the aid of an external pressurisation system different differential pressures acting at the vapor phase of the outer glass cylinder have been applied to move the liquid hydrogen meniscus inside the inner glass cylinder towards the double screen.
During all tests wall temperatures at the outer glass cylinder and at the inner glass cylinder at the height of both screens have been measured. In addition to that the differential pressure of the hydrogen vapor phases between the inner and outer glass cylinder has been recorded as well as the absolute pressure of the hydrogen vapor phase at the inner glass cylinder. Using a laser for illumination and an endoscope with connection to a CCD camera, videos could be recorded to track the liquid movement.
The experimental results confirm the predicted governing physical phenomena. In addition to that, influences due to evaporation and condensation have been observed.
Characteristic frames at certain time steps recorded during one of the 14 ground tests are denoted in the upper row of Fig. 3. The associated recorded frames during one drop tower experiment are shown in the lower row of Fig. 3 also at characteristic time steps.
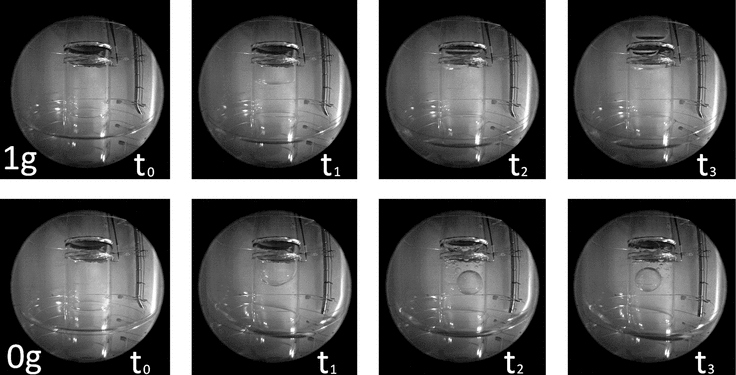
Publications
Michael Conrath, Nicolas Fries, Ming Zhang, and Michael E. Dreyer. Radial capillary transport from an infinite reservoir. Transport Porous Med., 84(1):109–132, 2010.
Michael Conrath and Michael E. Dreyer. Gas breakthrough at a porous screen. Int. J. Multiphas. Flow, 42:29–41, June 2012.
Michael Conrath, Yulia Smiyukha, Eckart Fuhrmann, and Michael Dreyer. Double porous screen element for gas-liquid phase separation. Int. J. Multiphas. Flow, 50:1–15, April 2013.
Acknowledgement
The project under the grant number 50RL1621 is funded with means of the German Federal Ministry for Economic Affairs and Energy through the German Aerospace Center (DLR e.V.).
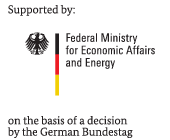