Space Exploration – Leaving Planet Earth
Some international space agencies want to send humans to the surface of the Moon within the next 20 years, and later to Mars as well. After several decades, there are again rockets powerful enough to transport astronauts outside the Earth's orbit – an important step to reach far away planets. But before we can make space exploration a reality, many important questions remain to be answered. At ZARM scientists are looking for answers: How can we increase the range of a space transporter and ensure the highest possible safety on board? How to accommodate a crew on the Moon or Mars once they arrived? How can the astronauts be supplied with food and fuel without replenishment from Earth?
The Mars habitat "MaMBA"
When we travel to other planets, we have to consider that we will be confronted with an environment that is hostile to humans. And, of course, there is no accommodation for a long-term stay. Therefore, so-called habitats are needed in which we can live and work.
Accross the globe, numerous test habitats have already been developed for research by scientists. However, these are only designed for use on Earth and to investigate organizational and psychological issues of an astronautic crew. There is still no habitat design that could actually withstand the extreme conditions on Moon or Mars.
In the MaMBA (Moon and Mars Base Analog) project at ZARM, scientists designed and constructed a habitat for long-term stays on Moon and Mars. The concept consists of six living and working spaces, called modules, each of which fulfils different functions. The laboratory module is a central place where people work together during the day. For this module a full-size mock-up has already been constructed at ZARM, with complete laboratory equipment, exactly as it could be used within the habitat on Moon and Mars.
Similar to a Tiny House, the aim is to adapt the equipment as best as possible to the limited space available. After all, the more compact the construction, the lower the significant costs of transporting the components from Earth to the Moon or Mars. The mock-up of the laboratory module has already been used for several simulations in which scientists have worked on their current research projects for a week at a time.
Dangers from inside and outside: protecting the astronauts
When developing a habitat, safety is paramount, so developers need to anticipate various scenarios of potential hazards within the modules. Should a life-threatening situation arise within a module, for example if chemicals leak or a fire breaks out, the astronauts need to be able to escape to unaffected modules. The damaged module is sealed off and the connection to the other ones interrupted. Accordingly, two independent life-support systems are provided for the entire habitat, so that a back-up is always available.
There is also a constant danger looming outside: Unlike on Earth, there is no sufficiently protective atmosphere on Moon or Mars, which places special demands on the astronauts' habitat. The habitat must protect the inhabitants from space radiation, temperatures and the vacuum outside. In addition, there may be threats from meteorite impacts or, especially on Mars, sandstorms. These dangers are mitigated by building a meter-thick protective shell around the actual habitat from the regolith available on site.
Psychological aspects are also taken into account when designing the habitats. Because when people feel comfortable in their environment, they can also work better. On a Moon or Mars mission, astronauts live in one place for a long time. Here they are around the same people every day and they have to manage to cooperate efficiently. It is not possible to go out for a breather to take your mind off things or go for a run, as on Earth. In addition, from Mars, contact with family and friends on Earth is limited by the long signal transit times. An important goal in the design of the habitat is therefore to create an environment that is as homely as possible and does not represent an additional burden. On the contrary, it should even help to make the transition to life on another planet easier. An example of a structural measure that is specifically intended to help against claustrophobic feelings is the generous ceiling height: in the communal and leisure modules, it is planned to be about five meters.
MaMBA in pictures
The two-storey laboratory module is the heart of the habitat. The scientists will spend a lot of time there to advance their research. On the lower level of the laboratory module, the scientists can do their research and on the upper level, there is space for storing material or equipment.
Modular equipment, designed for maximum flexibility, allows the scientists to work comfortably within the limited working space. Integrated into the outer wall of the module is a tank for the propagation of cyanobacteria, which, among other things, produce oxygen and are thus part of the life-support system.
Cyanobacteria – blue-green super talents
A basic requirement for survival on alien planets is that the few materials brought from Earth must be used efficiently to provide equipment and food for astronauts on Moon and Mars.
When humans go to Mars, we will need to provide the crew with large amounts of consumables: food, water, oxygen, and sometimes medication. And if our presence there is to be sustainable, all that cannot come from Earth, the costs and risks of resupply missions would be too high. The aim of the research on microbiology at ZARM is to create a self-sufficient ecosystem that is able to generate all the necessary consumables from local resources in order to be able to live independently on the red planet. This is where the blue-green cyanobacteria could soon play a key role.
For us, cyanobacteria are mainly known as blue-green algae in swimming lakes. The unwelcome summer guests do not enjoy a good reputation, because they often cause bathing restrictions. In too high a concentration, the bacteria become dangerous for humans. Allergies can be triggered by skin contact or stomach problems or diarrhea can occur when toxic water is swallowed.
However, their versatility makes microorganisms true superheroes for space missions. Like plants, they perform photosynthesis, produce oxygen, and form biomass. This can be a vital advantage for survival in an environment poor of oxygen and other ressources like on Mars. In short: cyanobacteria are real super talents.

LASM in pictures
In the Laboratory for Applied Space Microbiology (LASM) at ZARM, research is being conducted into which atmospheric conditions optimally promote the growth of these microorganisms. For this purpose, Atmos (Atmosphere Tester for Mars-bound Organic Systems) - an atmosphere-controlled vacuum photobioreactor - has been developed here, with which microorganisms can be cultivated and propagated outside their natural environment.
With the help of Atmos, the research team has been working to determine what atmospheric conditions would support the growth of cyanobacteria, while also improving technical feasibility on Mars. In Atmos, the proportions of the gases and the atmospheric pressure were changed in various runs and the corresponding development of the bacteria was observed. The goal of the investigations was to get as close as possible to the Martian atmosphere while still maintaining strong growth of the cyanobacteria.
Artificial Photosynthesis – Oxygen production in space
For us on Earth it is very normal that we do not think about where oxygen comes from: plants and cyanobacteria produce it for us for about 2.3 billion years. It’s just so fantastic that we have nature! But what when we are in space?
We are used to the phenomena that in less than a second, natural photosynthesis in plants magically transforms carbon dioxide and water into glucose and oxygen using sunlight – with oxygen being only a ‘waste product’ of this biological process.
It is easy to imagine that we need to solve a key problem for all space missions to the International Space Station (ISS), Mars or Moon: how do we produce sustainably and efficiently oxygen (and an artificial atmosphere) for long periods of time? How do we recycle carbon dioxide exhaled by astronauts? Currently, oxygen and hydrogen are produced through water electrolysis in the so-called “oxygen generator assembly” (OGA) on the ISS. The energy required for the reaction is fed into the reactor from incoming sunlight via solar panels outside the space station. That sounds great – it sounds like everything works already!
Not quite. The near-absence of buoyancy caused by microgravitation on the ISS hinders the detachment of gas bubbles from electrodes in the electrolyser and the produced gases (oxygen and hydrogen) can only be detached and utilised through rotation. This requires a lot of energy: 1.5 kW out of the 4.6 kW used by the entire Environmental Control and Life Support System (ECLSS) on the ISS is used by the OGA to produce oxygen for crew members. For long-term missions to the Moon or Mars, life-support systems that must rely on sunlight as their sole energy source must become much more efficient.

How we mimic natural photosynthesis
Scientists at ZARM work on the development of alternative methods to produce oxygen and other chemicals (such as hydrogen) efficiently and sustainably for long time periods at minimal energy input: Their aim is to mimic the key processes of natural photosynthesis to design so-called “artificial photosynthesis devices”. Instead of chlorophyll, they utilise semiconductors to absorb light and coat them with electrocatalysts to integrate the processes of light absorption, charge separation and catalysis. This allows us to potentially produce oxygen and hydrogen using less weight and volume than traditional electrolysers.
Semiconductors and electrocatalysts can moreover be energetically altered and optimised for a large variety of reactions: the researchers not only work on the realisation of an autonomic oxygen and hydrogen production device in microgravity, but also on the (photo-)electrochemical reduction of carbon dioxide and the production of other chemicals such as urea (a fertiliser) and more complex precursors for pharmaceuticals.
Fire on Board
In astronautic missions, the protection of human life is always the first priority. Fire safety on board a spacecraft is therefore an important issue. Due to the weightlessness, however, a completely different approach is required than on Earth.
A fire on board a spacecraft is one of the most dangerous scenarios in space missions. There are hardly any options for getting to a safe place on board or escaping from a spacecraft. It is therefore crucial to understand the behavior of fires under these special conditions.
ZARM researchers has been conducting experiments on the propagation of fires in weightlessness since 2016. In their experiments the environmental conditions are similar to those on the ISS – with an oxygen level in the breathing air and an ambient pressure similar to that on Earth, as well as forced air circulation. These experiments have shown that flames behave completely differently in weightlessness than on Earth: A fire burns with a smaller flame and spreads more slowly, which means it can go unnoticed for a long time. However, it burns hotter and can therefore also ignite materials that are basically non-flammable on Earth. In addition, incomplete combustion can produce more toxic gases. And most suprisingly, a flame tends to spread in the opposite direction to the air flow. Accordingly, trying to blow out a flame on a spaceship would be a very bad idea!
Why do flames behave differently in weightlessness?
On Earth, the layers of air surrounding a flame flow upwards. Gravity takes care of this: the layers of air heat up and expand, thus they become lighter and rise. As new air flows in from below, oxygen is permanently supplied to the flame.
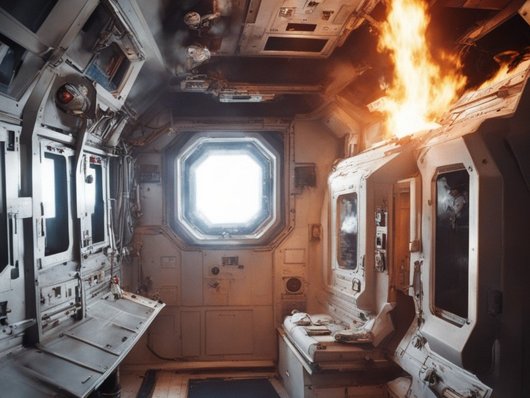
Combustion under zero gravity behaves differently. Without the surrounding air flow, the flame takes on a spherical shape. The combustion gases move out of the flame slowly, and hardly any oxygen can reach the flame. As a result, the flame burns hotter and smaller and goes out on its own after some time.
Despite a supposedly less active fire development in weightlessness, a fire on a space station can become a big problem. Due to the many technical devices on board, there is generally a high risk of fire on a space station. If they are overloaded, they can start a fire. Ventilation systems that cause a small draft of air can keep a fire alive that would normally go out on its own. This behavior was also observed in experiments, where plexiglas probes were ignited in space: After the planned observation period of the fire, the ventilation was switched off to extinguish the fire. Unexpectedly, the temperature remained very high for a long time, so that switching on the ventilation again reignited the fire. Even after seven hours of cooling, 60 degrees Celsius were still measured on the sample.
Fire experiments in weightlessness
In 2021, a team of 25 international scientists used the Northrop Grumman CYGNUS supply vehicle for the ISS to study the behavior of fires in space. During the SAFFIRE (Spacecraft Fire Safety Demonstration) experiment, the team collected data material, and took thousands of individual photos, documenting the combustion of a five-centimeter-wide, one-centimeter-thick Plexiglas sample.
A current series of experiments with very thin plexiglass foils is being carried out under weightlessness in the Drop Tower Bremen. Here, ZARM scientists are observing the propagation of flames after igniting the samples to investigate how the fire reacts when one of the three parameters – ambient pressure, oxygen content and flow velocity – is changed in different proportions. The reason for this is that future space missions are currently being planned with modified atmospheric conditions, e.g. lower pressure and higher proportions of oxygen in the breathing air for the crew. This can have dangerous consequences in the event of a fire.
Refueling Stations in Space
For many decades, mankind has been striving to explore space not only from Earth, but also in orbit with the help of spacecraft. So far, there are natural limits to this exploration. One of them is the fuel supply.
In general, missions with an astronautic crew are always designed for an outward and return flight. Therefore, the spacecraft may only move so far away that it can return with the fuel it has on board. This makes it impossible to jump too deep into space. But refuelling stations or fuel depots in space could extend the flight range of spacecraft into deep space.
However, refueling in space is also relevant for non-astronautic missions. If you want to launch a fully fueled spacecraft into space, the rocket launch – the moment to escape the Earth's gravitational pull – alone consumes enormous amounts of fuel, depending on the weight of this payload. An alternative would be to launch the spacecraft designed for long exploration missions with an empty tank and refuel it in space. The empty tank saves weight and thus fuel for the launch vehicle. The transport of the fuel depot on the one hand and of the fuel to the depot on the other hand can be accomplished with pure cargo rockets (i.e. without crew). These rockets are subject to lower safety requirements than rockets that are approved for passenger transport and are therefore allowed to transport larger amounts of fuel. This is more efficient and saves costs.
On Earth, we have a dense network of fuel stations to ensure the availability of fuel almost everywhere. Similarly, installing refueling stations in orbit or on the surface of Moon or Mars could significantly increase our range of motion.
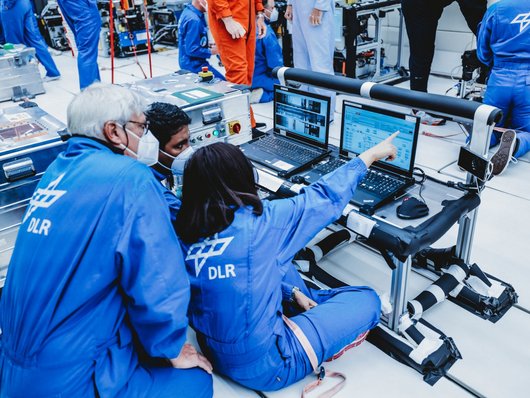
Concepts for propellant filling-stations
An important requirement for this ambitious project is the controlled handling of fluids in space – a topic that the "multiphase flows" research group at ZARM has been working on for many years. Their current project, "Zero Boil-Off Tank Experiment – Refuelling and Transfer", is investigating the filling of empty or partially empty spacecraft tanks with hydrogen or methane in order to be able to realize future filling stations in space. In concrete terms, it is about the problems of pressurizing a donor tank as well as cooling and filling a receiver tank with special focus on the given acceleration and temperature.